Abstract
Objective: The aim of this study was to assess the diagnostic
potential of 18F-FDG Positron Emission Tomography imaging in the
evaluation of patients with solitary pulmonary nodules, by comparing the
diagnostic criteria in single time point imaging method to two different diagnostic
criteria in dual-time-point imaging.
Methods: This retrospective study was conducted in the Hospital
of the University
of Pennsylvania, and data
was collected and analyzed in the period from September 2005 to March 2006,
from the pooled hospital studies for the last eight years. Two hundred sixty five
patients were included (161 men, 104 women, age range: 41–92 years). All had solitary
pulmonary nodules on computed tomography, and the diagnosis was confirmed by
biopsy or by follow up computed tomography. All 265 patients underwent whole
body FDG PET scan, and 255 of them had PET scan two time points. The maximum standardized
uptake values of nodules were calculated for both time points. On
single time point imaging we set the maximum standardized uptake value of 2.5
as a cutoff criterion for malignancy. On dual time point imaging, first criterion
of malignancy was set as any increase in the maximum SUV from the first to
second time point. The second criterion was set as either no change or increase
in the maximum standardized uptake value between the two time points. Sensitivity,
specificity and accuracy were calculated for the three methods by using the
biopsy results and clinical follow up as gold standard.
Results: Biopsy and follow-up revealed 72 patients
with malignant lung nodules, whereas 193 patients had benign nodules.
Single time point imaging with a threshold maximum standardized
uptake value of 2.5 had a sensitivity, specificity and accuracy of 63%,
92% and 85% respectively. On dual-time-point imaging, for the initial criterion
for malignancy, the sensitivity, specificity and accuracy were 81%, 95% and 91%
respectively. On dual time point imaging, for the second criterion for
malignancy, the sensitivity, specificity and accuracy were 92%, 93%, and 92%
respectively.
Conclusion: Dual-time-point FDG PET imaging using both criteria
has higher sensitivity, specificity and accuracy compared to single time
imaging. Dual-time-point FDG PET imaging should be included in the clinical
workup of patients with pulmonary nodule.
Key Words: Dual-time-point
imaging, FDG-PET, Solitary pulmonary nodule
JRMS
August 2008; 15(2): 6-14
Introduction
Solitary pulmonary nodules are defined as focal, round
or oval areas of increased opacity in the lung that measure less
than three cm in diameter.(1,2,3) An estimated
150,000 solitary pulmonary nodules are detected annually in the
United States and are often discovered incidentally at chest radiography
or computed tomography (CT).(3,4) These nodules
are caused by a variety of disorders including neoplasms, infection,
inflammation, and vascular and congenital abnormalities. Although
most solitary pulmonary nodules have benign causes, 30%–40% of these
nodules are malignant.(4-6)
18F-FDG
has established role in oncology, which includes initial diagnosis,
staging, and therapeutic follow-up studies.(7,8) Despite its proven utility, the
application of PET is limited by its variable sensitivity and
specificity estimates. One of the main reasons for this limitation
is that many inflammatory lesions also have elevated 18F-FDG uptake
in PET, leading to false-positive results.(9,10) On the other hand, some types of
cancers, for example, carcinoid tumor and bronchoalveolar carcinomas, have
low 18F-FDG uptake below the diagnostic threshold for 18F-FDG
uptake in malignant lesions.(11,12)
A maximum standardized uptake value (SUV) of 2.5 as a
cutoff criterion for malignancy has been used for diagnosing
pulmonary malignancies with 18F-FDG PET.(13-20) However, one study indicated that the
sensitivity of this SUV cutoff was lower than that of visual
assessment.(21)
Some authors have recommended using visual evaluation rather than
the SUV for small solitary pulmonary nodules,(22) suggesting that the classical SUV
criterion of 2.5 is inappropriate for diagnosing malignancies with
low 18F-FDG uptake.(21,23)
Studies have shown that the uptake of 18F-FDG continues
to increase in malignant tumors for several hours after 18F-FDG
injection.(10,12)
It has been deduced that this difference in the time course of 18F-FDG
uptake could be used to improve the ability of PET to distinguish
benign lesions from malignant lesions. Preliminary studies have been
performed using FDG PET with dual-time-point imaging on head and
neck cancers, breast cancer and malignant lung lesions. Those results demonstrated
significant improvement in the diagnostic accuracy of FDG PET scan.(12,21-25)
On the basis of the promising results from
dual-time-point imaging research, the present study was undertaken
to assess whether dual-time-point acquisition can improve the
diagnostic utility of PET in Solitary Pulmonary nodules.
Methods
This retrospective study was conducted in the Hospital
of the University
of Pennsylvania, and data
was collected and analyzed in the period from September 2005 to March 2006,
from the pooled hospital studies for the last five years. Two hundred sixty five
patients (161 men, 104 women; mean age 67 years; age range: 41–92 years)
were included in this retrospective analysis. All patients had suspected solitary
pulmonary nodules detected by CT. All our study patients had 18F-FDG
PET and CT scanning acquired in two different occasions, with a time gap (0-35
days). All patients underwent whole body
PET scans, and 255 patients were examined twice: initial whole-body
imaging followed by a second scan for the chest only. Informed
consent was obtained from all
patients. At the time of 18F-FDG injection all patients
had fasted for at least four hours and had blood sugar levels of
<150 mg/dL.
Image acquisition for the whole-body scan started
at a mean time point of 60 minutes after injection of 2.52
MBq/kg of body weight. This first scan (scan A) included neck,
thorax, abdomen, pelvis and upper thighs. It consisted of four or five
emission frames of 25.6-cm length with an overlap of 12.8 cm
covering an axial length of 64–76.8 cm, including six to seven beds
and duration of the scan was 18-21 minutes. A second emission scan of the
thorax only (scan B) was acquired on 255 patients at a mean time of
110 minutes after tracer injection (range 100–120 min), including two
beds and duration of scan was ranging between six minutes. A transmission scan
was obtained with both sets of images for attenuation correction. Image reconstruction was performed with
an iterative ordered-subsets expectation maximization algorithm with
four iterations and eight subsets. Attenuation-corrected images were
obtained by applying transmission maps, which were acquired after 18F-FDG
injection with a 137Cs source interleaved with the
emissions scans.
Regions of interest (ROIs) were overlaid onto the
lesions on fully corrected PET images of scans A and B axial slices
(Fig. 1). This was achieved by direct visual assessment of the
lesion position on the CT scan and subsequent identification of the
corresponding area on PET scans A and B.
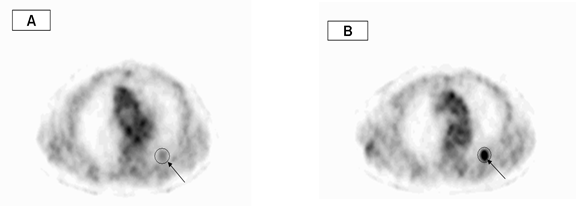
SUV=1.8 SUV=3.2
Fig.1. An axial slices region of interest (ROI) were placed
around the lesion on first time (A) and second time (B) images, in order to
calculate the maximum SUV
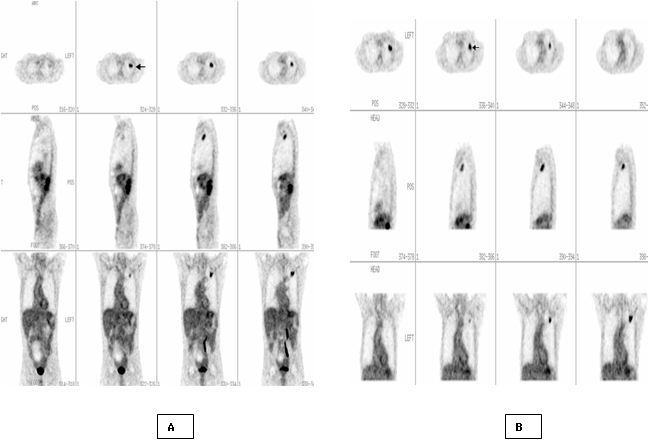
Fig.
2. Dual-time-point
FDG PET imaging of 60 years old man with 1.8 cm solitary pulmonary nodule in
the left lung on transverse, sagittal and axial slices. First time whole body
image (A) shows a focal area with FDG upatke (arrow) and with SUV= 2.5. Second
time image of the chest(B) shows more prominant FDG upatke with SUV=2.9.
Pathological diagnosis of this nodule was moderately differentiated
adenocarcinoma
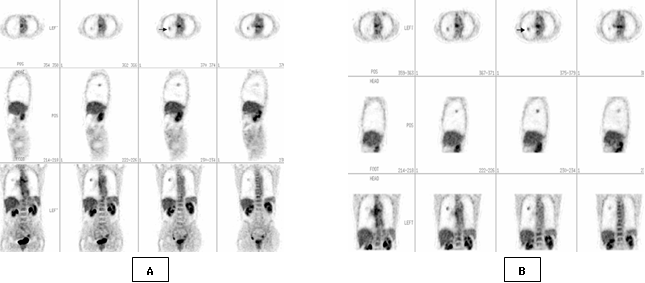
Fig. 3. Dual-time-point FDG PET imaging of 65 years old
male patient with 1.4cm pulmonary nodule in the right lung on
transverse, sagittal and axial slices . First time whole body image (A) shows
an area with FDG upatke ( arrow), with SUV= 1.8 and second time image of the
chest(B) with SUV =2.3. Pathological diagnosis of this nodule was bronchioloalveolar
adenocarcinoma
In
tumor lesions that extended over several slices in the craniocaudal direction,
the ROI was placed in the midportion of the lesion where the maximal
SUV was measured. If no discernible uptake was present on either PET
scan, ROIs were drawn in the presumed location that corresponded
best with that of the radiographic density. The maximum Standardized
uptake value (SUV) of the lung lesions were calculated from scan A and scan B
according to the following standard formula: Mean ROI activity (MBq/g) /
[Injected dose (MBq)/ Body weight (g)].
We used three criteria in the assessment of pulmonary
nodules; first criterion is the classical single time imaging using SUV of 2.5
as cut off criterion for malignancy. We adopted two criteria on dual time point
imaging; first criterion was set as any increase in SUV between the first and
second scans as a criterion for malignancy, while second criterion
was set as any increase or no change in SUV between the first and second scan
as criterion for malignancy. All nodules with no FDG uptake that had SUV=lung
background activity were considered as negative for malignancy in all criteria.
Benign or malignant diagnosis of the nodules was
established using biopsy or clinical follow up data. The sensitivity,
specificity, accuracy, negative predictive value (NPV) and positive predictive
values (PPV) for the three criteria were calculated.
Results
Of the 265 nodules included in this study, 72 (27%)
proved to be malignant and 193 (73%) benign. The sizes of these 265
nodules were as follows: 32 nodules <1 cm in diameter, 57 nodules
1-1.5 cm and 176 nodules 1.6-3 cm. Diagnoses of 158 nodules were
decided to be benign according to the clinical follow up data.
On single time point imaging, 60 nodules had SUV≥2.5 (45
malignant and 15 benign), 205 nodules had SUV<2.5 (27 malignant and 178
benign). While 119/205 nodules did not show any visually apparent FDG uptake in
the first scan and SUVs of those lesions were equal to the lung background.
On dual-time-point imaging, 60 nodules had increased
in SUV between scan A and scan B (50 malignant and 10 benign), 70 nodules had
drop in SUV (three malignant and 67 benign) and 11 nodules had no change in SUV
(seven malignant and four benign). One hundred and fourteen nodules had no FDG
uptake on scan A and B and the calculated SUV= lung background (two malignant
and 112 benign). Figures 2 & 3 show dual-time-point imaging in two patients
with malignant pulmonary nodules, including SUV change and histopathology diagnosis.
When assessing the diagnostic value of the first
emission scan by applying an SUV threshold of 2.5
for
separating benign from malignant lesions, sensitivity, specificity
and accuracy were 63%, 92% and 84% respectively. When applying this criterion
to small nodules of less than 1cm, the values were 50%, 90% and 75%
respectively compared to 65%, 93% and 85% for larger nodules.
When any increase in SUV between the first and second
scans was applied as a criterion for malignancy, the sensitivity,
specificity and accuracy were 83%, 95% and 91% respectively. When any increase
or no change in SUV between the first and second scans criterion was applied,
the values were 92%, 93%, and 92% respectively. Table I and II shows SUV1, SUV2
and histopathological diagnoses for benign and malignant lung nodules. Table III
shows the sensitivity, specificity, accuracy, NPV and PPV in the three criteria
used in our study according to single time SUV and dual time change.
Discussion
In addition to visual assessment of the metabolic
activity of the nodules, measurement of the SUV for the
semiquantitative assessment of 18F-FDG uptake in
pulmonary lesions has proven to assist in differentiating between
malignant and benign nodules.(13-20) Several reports
consider it to be a simple and useful tool for this purpose, and
most publications conclude that a threshold value of 2.5 is optimal
for obtaining a high sensitivity while maintaining a good
specificity.(13-20) However, several reports and
observations on the day-to-day clinical practice indicate that a
significant degree of overlap exists between the uptake values of
benign and malignant lesions.(16-18)
Certain inflammatory lesions, including granulomatous processes,
fungal infections, or bacterial infections, can be noted with SUVs
of >2.5,(15,26) thereby limiting specificity of
this method. Most inflammatory lesions would fall
below the 2.5 SUV thresholds, whereas the majority of malignant
lesions would have high SUVs. However, small malignant
lesions may have under
estimated
SUV due to partial volume effect and limited resolution of PET scanner.(27-28)
On the other hand, carcinoid tumor and bronchoalveolar carcinomas can have low
levels of 18F-FDG uptake, and the SUV in such tumors may fall below
the 2.5 limit for malignancy in this criterion.(11,12) Those
factors have potential effect on the diagnostic accuracy of using this
criterion, because it can lead to misinterpretation of malignant lesions into
benign ones.
In our study, when adopting the SUV ≥ 2.5 as criterion
for malignancy, the sensitivity, specificity and accuracy were 63%, 92% and 84%
respectively. Our results have lower sensitivity and comparable specificity
compared to those published in the literature.(13-18) When applying this criterion to small
nodules of less than 1cm, the values were 50%, 90% and 75% compared to 65%, 93%
and 85% for larger nodules. This can show the
limitation of this criterion induced primarily by underestimation of SUV due to
impact of partial volume effect.
Dual-time-point FDG PET imaging was suggested as
discriminator of benign and malignant diseases, with images being
obtained at one and two hours after the administration of 18F-FDG.
Hustinx et al.(9)
had acquired dual time point imaging for head and neck tumors, and
he used a threshold of 10% increase in measured values. He reported higher
sensitivity (100% vs. 80%), while maintaining an excellent specificity
(89% vs. 94%), than that obtained from a single image acquisition
using the usual SUV threshold method. Zhuang et al.(10)
found that malignant lesions showed a significant increase in SUV
over time and that benign lesions showed a decrease over time. Lodge
et al.(26) came to a similar conclusion in a study of
29 patients with various benign and malignant soft-tissue masses. Rakesh et
al.(25) had applied dual time point imaging in breast
cancer, and found that breast malignancies show increasing FDG uptake with
time, whereas the uptake of 18F-FDG in inflammatory
lesions and normal breast tissues decreases over time.
Table I. SUV1 and SUV2 in malignant lung nodules
SUV1
|
SUV2
|
Final diagnosis
|
SUV1
|
SUV2
|
Final diagnosis
|
2.5
|
2.6
|
Poorly differentiated
adenocarcinoma
|
2.1
|
2.3
|
Bronchioloalveolar adenocarcinoma
|
1
|
1.6
|
Differentiated
adenocarcinoma
|
4.2
|
4.8
|
Lung adenocarcinoma of
moderately differentiated,
|
1.7
|
2.4
|
Metastatic adenocarcinoma of
breast
|
2.5
|
2.8
|
Well-differentiated
pulmonary adenocarcinoma
|
2.9
|
4
|
Poorly differentiated adenocarcinoma
|
2.7
|
3
|
Moderately differentiated
adenocarcinoma
|
7.5
|
10
|
Squamous cell carcinoma
|
1.3
|
1.5
|
Poorly differentiated
adenocarcinoma
|
3.1
|
4.1
|
moderately differentiated
adenocarcinoma
|
5.8
|
6.5
|
Poorly differentiated
adenocarcinoma
|
3.1
|
4.1
|
Small cell lung cancer
|
1.6
|
1.8
|
Mucinous adenocarcinoma Colon metastasis
|
1
|
1.3
|
Moderately differentiated
adenocarcinoma
|
4.3
|
4.8
|
Adenocarcinoma, moderately
differentiated
|
3.1
|
4
|
Poorly differentiated
adenocarcinoma
|
2
|
2.3
|
Poorly differentiated
adenocarcinoma
|
1.8
|
2.3
|
Carcinoma of neuroendocrine
origin, possible small cell lung cancer
|
4.8
|
5.4
|
Metastatic esophageal adenocarcinoma
|
2.9
|
3.7
|
Poorly differentiated
adenocarcinoma
|
2.7
|
3
|
Well diff. adenocarcinoma
|
5.9
|
7.5
|
Well-differentiated
adenocarcinoma
|
6.1
|
6.4
|
Well-differentiated
adenocarcinoma
|
4.6
|
5.8
|
Bronchioloalveolar adenocarcinoma
|
6
|
6.5
|
Poorly differentiated
adenocarcinoma,
|
5.4
|
6.8
|
Squanous cell lung cancer
|
17.7
|
17.7
|
Moderately differentiated
adenocarcinoma
|
2.8
|
3.5
|
Metastatic transitional cell
ca of bladder
|
5.4
|
5.4
|
Moderately differentiated adenocarcinoma
|
1.2
|
1.5
|
Moderate-poor differentiated
Squamous cell carcinoma
|
3.4
|
3.4
|
Moderately differentiated
adenocarcinoma
|
1.2
|
1.5
|
Moderately differentiated
adenocarcinoma
|
2.2
|
2.2
|
Differentiated
adenocarcinoma
|
1.8
|
2.2
|
Well differentiated adenocarcinoma
|
2.3
|
2.3
|
Bronchioloalveolar adenocarcinoma
|
7.4
|
9
|
Squamous cell lung cancer
|
2
|
2
|
Metastatic melanoma
|
4.7
|
5.7
|
Poorly differentiated adenocarcinoma
|
1.7
|
1.7
|
Bronchoalveolar
adenocarcinoma
|
2.1
|
2.5
|
Poorly differentiated adenocarcinoma
|
2.6
|
2.5
|
Metastatic germ cell tumor
|
2.1
|
2.5
|
Poorly differentiated
adenocarcinoma
|
2.8
|
2.6
|
Metastatic breast cancer
|
1.1
|
1.3
|
Moderately differentiated
adenocarcinoma
|
2.8
|
2.6
|
Atypical carcinoid
|
1.2
|
1.4
|
Moderately differentiated
adenocarcinoma
|
1.4
|
1.3
|
Carcinoid
|
2.6
|
3
|
Adenocarcinoma
|
2.5
|
2.3
|
Adnocarcinoma
|
1.3
|
1.5
|
Bronchioloalveolar adenocarcinoma
|
0.5
|
0.7
|
carcinoid
|
3.3
|
3.8
|
Poorly differentiated adeno
carcinoma
|
15
|
|
Poorly differentiated
adenocarcinoma
|
2.7
|
3.1
|
Poorly differentiated
non-small cell carcinoma
|
13.7
|
|
Metastatic vocal cord tumor
|
2.7
|
3.1
|
Poorly differentiated
Adenocarcinoma
|
10.6
|
|
Poorly differentiated
squamous cell carcinoma
|
2.7
|
3.1
|
Metastatic transitional cell
carcinoma
|
7.7
|
|
Non Small cell cancer, Large
cell cancer
|
7
|
8
|
Poorly differentiated
adenocarcinoma, with focal sarcomatoid features
|
4.4
|
|
Bronchioloalveolar
adenocarcinoma
|
2.3
|
2.6
|
Moderately differentiated
adenocarcinoma
|
3.4
|
|
Poorly differentiated
adenocarcinoma
|
1.7
|
1.9
|
Mucinous adenocarcinoma
metastasis of colon
|
3
|
|
Baldder adenocarcinoma mets
|
1.8
|
2
|
Bronchioalveolar
adenocarcinoma
|
2.9
|
|
Moderately differentiated
adenocarcinoma
|
2
|
2.2
|
Carcinoid
|
2.8
|
|
Moderate to poorly
differentiated adenocarcinoma
|
2
|
2.2
|
Small cell cancer
|
2.5
|
|
Metastatic melanoma
|
Table
II. SUV1 and SUV2 in benign lung
nodules
SUV1
|
SUV2
|
Final diagnosis
|
SUV1
|
SUV2
|
Final diagnosis
|
2.6
|
2.8
|
Sarcoidosis
|
2.3
|
2.1
|
Decreased
in size by CT
|
3.5
|
4.1
|
Inflammation
|
1.9
|
1.4
|
Inflammation
|
2.6
|
2.3
|
Granuloma
|
1.6
|
1.2
|
decreased
size by CT
|
2.7
|
2.1
|
Inflammatory
|
1.6
|
1.2
|
Granulaoma
|
2.9
|
2..5
|
Mycobacterium
Infection
|
2.5
|
2.1
|
Inflammation
|
3.2
|
1.6
|
Inflammation
|
1.4
|
1.3
|
Stable
on F/U CT
|
2.5
|
2.1
|
Inflammation
|
2.3
|
2.4
|
Histoplasmoma
with granuloma
|
2.6
|
2.7
|
Chondromatous
hamartomas
|
2
|
1.6
|
Inflammation
|
2.8
|
2.4
|
Stable
on CT
|
2.3
|
1.9
|
Stable
|
2.6
|
2.6
|
Resolved
on CT
|
1.5
|
1.6
|
Inflammation
|
2.7
|
2.2
|
Inflammation
|
2.1
|
1.6
|
Stable
|
2.8
|
2.4
|
Fibrosis
|
1.8
|
2.4
|
Apical
sub pleural fibrosis
|
2.6
|
2.6
|
Inflammation
|
2
|
1.8
|
Resolved on CT
|
2.5
|
2.2
|
Stable
in PET F/U
|
2.1
|
1.7
|
Inflammation
|
2.6
|
2.3
|
Stable
on CT F/U
|
2.2
|
2
|
Inflammation
|
1.2
|
1.2
|
Inflammation
|
1.4
|
1.1
|
Stable
on CT
|
2.2
|
1.8
|
Resolved
CT
|
2.2
|
1.9
|
Noncaseating
Granuloma
|
2.1
|
2.1
|
Chronic
inflammation
|
2.1
|
2
|
Stable
on CT
|
1.4
|
1.4
|
Stable
on CT
|
1.8
|
1.6
|
Inflammation
|
2
|
1.7
|
Fibrosis
|
2.4
|
2.6
|
Granuloma
|
1.9
|
1.5
|
Resolved on CT
|
1.7
|
3.5
|
Inflammation
|
1.9
|
1.6
|
Inflammation
|
1.9
|
1.8
|
Stable
|
2.3
|
2.8
|
Inflammatory
|
2.4
|
2.5
|
Atypical
Mycobacterial Infection
|
1.7
|
1.6
|
Stable
in CT
|
1.1
|
0.9
|
Decreased
size
|
2.3
|
2.1
|
Inflammation
|
1.8
|
1.5
|
Granuloma
|
1.4
|
2.1
|
Granuloma
|
2.4
|
2.2
|
Inflammation
|
1.9
|
1.7
|
Hamartomas
|
|
|
|
Table
III. The results of statistical
analysis in three different methods used in the assessment of solitary
pulmonary nodules
Criteria
|
FN
|
FP
|
TP
|
TN
|
Sensitivity
|
Specificity
|
Accuracy
|
PPV
|
NPV
|
SUV ≥ 2.5
|
27
|
15
|
45
|
178
|
63%
|
92%
|
84 %
|
71%
|
89%
|
Any increase in SUV on Dual-time-point imaging
|
12
|
10
|
50
|
183
|
83%
|
95%
|
91%
|
83%
|
94%
|
Increase or no change in SUV on Dual-time-point
imaging
|
5
|
14
|
57
|
179
|
92%
|
93%
|
92%
|
78%
|
97%
|
Hamberg et al.(29) showed
that the usual scan start times of 45–60min lead to significant
underestimation of the true SUV because, in most tumors, 18F-FDG
uptake continues to rise beyond this period and typically does not
reach a plateau for several hours. In untreated tumors, 95% of the
plateau value was reached at 298 ± 42 min, with a range of 130–500
min.
We set two criteria for the assessment of pulmonary
nodules with dual-time-point imaging, in order to
decide which is going to give us the most accurate results when compared to
biopsy and clinical follow up. In our first criterion with any
increase in SUV between the first and second scans had sensitivity, specificity
and accuracy of 83%, 95% and 91% respectively vs. 63%, 92% and 84% for single
time point imaging with SUV ≥ 2.5 criterion. This criterion shows clear benefit
of dual time point imaging in improving the sensitivity while maintaining good
specificity. When the criterion was changed into any increase or no change
between the two scans, sensitivity, specificity and accuracy were 92%, 93% and
92% respectively. In this criterion there were seven malignant nodules which
did not show any change in SUV on dual-time-point imaging, and the
interpretation of which was changed from false negative into true positive. The
sensitivity of PET increased to 92% when the second dual-time-point criterion
was used vs. 63% in single-time-point PET. On the other hand, dual-time-point
imaging using the later criterion has a high negative predictive value for
malignant nodule (97%). This high negative predictive value
may allow us to wait and have a follow-up evaluation of the SPN after
a certain time interval of three or six months.
Matthies et al.(12) compared
single-time-point imaging and dual-time-point imaging with a cutoff
SUV of 2.5 and a 10% increase in SUV for malignancy in 36 pulmonary nodules,
which was a relatively small study group; the authors determined
that the sensitivity and specificity of the tests were 80% and 94%
(single) and 100% and 89% (dual), respectively. Although there is
clear benefit of dual-time-point imaging using this criterion, still in our
study there were 10 malignant lesions that did not reach the 10% increase in
SUV (drop in three and no change in seven), and this criterion can result in
misinterpretation of those lesions as benign.
Our results are in contrast to a study by Lowe et
al.(18) who assessed the change in SUV over
time in a cohort of 14 patients with pulmonary abnormalities (10
malignant, four benign). On the basis of measurement of the
signal-to-noise ratio, the best separation between benign and
malignant lesions occurred at 50 minutes after injection and no
improvement was seen at later time points.
This study included 57 FDG avid benign lung
nodules. Pathological diagnosis of FDG avid benign
lesions that had increase
or no change
in SUV included: inflammation, granuloma, histoplasmoma,
mycobacterial infection, and sarcoidosis. Also in the literature some benign
granulomatous lesions, such as sarcoidosis, aspergillosis, and
coccidiomycosis, have been reported to be 18F-FDG avid and to show
increasing uptake over time and producing false positive results.(20,30,31)
The limitation in the present study is that during
semiquantitative analysis with only a PET scanner, the ROI location
that corresponded to the lesion site was difficult to determine only
on PET images when the lesion was faint or presence of other FDG
avid benign lesions. Thus, we selected a nearby location by using
corresponding CT slices; this method would have produced some
inaccuracies in SUV measurements. This problem can be resolved by
using a PET/CT scanner, because the ROI location can be determined
easily by use of fused PET and CT images. However, the use of
dual-time-point imaging would add to diagnostic accuracy, especially
for small lesions that have lower SUVs, and in differentiating inflammation
from malignant lesions; this increase in diagnostic accuracy would
compensate for the extended length of each scan.
Conclusion
Dual time point FDG Positron Emission Tomography using
both criteria has higher sensitivity, specificity and accuracy compared to
single time imaging. Dual time point FDG Positron Emission Tomography should be
included in the clinical workup of patients with solitary pulmonary nodule.
References
1. Ost D, Fein A, Feinsilver S. Clinical practice. The solitary pulmonary nodule. N
Engl J Med 2003; 348(25): 2535-2542.
2. Chin
Y, Kyung L, Byung-Tae K, et al. Tissue
Characterization of Solitary Pulmonary Nodule: Comparative Study Between
Helical Dynamic CT
and Integrated PET/CT. J Nucl Med 2006; 47: 443-450.
3. Leef J, Klein I. The solitary pulmonary nodule. Radiol Clin North Am
2002; 40: 123-143.
4. Tan B, Flaherty K, Kazerooni E, et al.
The Solitary Pulmonary Nodule. Chest.
2003; 123: S89-S96.
5. Gurney J. Determining the likelihood of malignancy in solitary
pulmonary nodules with Baysian analysis. Part
I. Theory. Radiology 1993; 186: 405–413.
6. Patz
E. Evaluation of Focal Pulmonary
Abnormalities with FDG PET. Radiographics 2000; 20: 1182-1185.
7. Rohren E, Turkington T, Coleman R. Clinical applications of PET in oncology. Radiology
2004; 231: 305–332.
8. Kumar R,
Bhargava P, Bozkurt M, et al. Positron emission tomography imaging in evaluation of cancer patients. Indian
J Cancer 2003; 40: 87–100.
9. Hustinx R, Smith RJ, Benard F, et
al. Dual time point
fluorine-18 fluorodeoxyglucose positron emission tomography: a potential method
to differentiate malignancy from inflammation and normal tissue in the head and
neck. Eur J Nucl Med 1999; 26: 1345–1348.
10. Zhuang
H, Pourdehnad M, Lambright ES, et al. Dual time point 18F-FDG PET imaging for
differentiating malignant from inflammatory processes. J Nucl Med 2001; 42:
1412–1417.
11. Buck A,
Schirrmeister H, Kuhn T, et al. FDG uptake in breast cancer: correlation with biological and clinical
prognostic parameters. Eur J Nucl Med Mol Imaging 2002; 29: 1317–1323.
12. Matthies A, Hickeson M, Cuchiara A, et
al. Dual-time-point 18F-FDG
PET for the evaluation of pulmonary nodules. J Nucl Med 2002; 43: 871–875.
13. Al-Sugair A, Coleman R. Applications of PET in lung cancer. Semin Nucl Med 1998; 28: 303–319.
14. Kubota
K, Matsuzawa T, Fujiwara T, et al. Differential diagnosis of lung tumor with positron emission tomography:
a prospective study. J Nucl Med 1990; 31: 1927–1932.
15. Gupta N,
Frank A, Dewan N, et al. Solitary pulmonary nodules: detection of malignancy with PET with
2-[F-18]-fluoro-2-deoxy-D-glucose. Radiology 1992; 184: 441–444.
16. Patz E,
Lowe V, Hoffman J, et al. Focal
pulmonary abnormalities: evaluation with F-18 fluorodeoxyglucose PET scanning. Radiology
1993; 188: 487–490.
17. Dewan N, Gupta N, Redepenning L, et
al. Diagnostic efficacy of
FDG-PET imaging in solitary pulmonary nodules: potential role in evaluation and
management. Chest 1993; 104: 997–1002.
18. Lowe V, Fletcher J, Gobar L, et al. Prospective investigation of positron emission
tomography in lung nodules. J Clin Oncol 1998; 16; 1075–1084.
19. Präuer H, Weber W, Römer W, et al. Controlled prospective study of positron emission
tomography using the glucose analogue [18F] fluorodeoxyglucose in
the evaluation of pulmonary nodules. Br J Surg1998; 85: 1506–1511.
20. Kotaro
H, Yoshimichi U, Hiroyasu S, et al. Fluorine-18-FDG PET imaging is negative in bronchioalveolar carcinoma. J
Nucl Med 1998; 39: 1016-1020.
21. Nomori
H, Watanabe K, Ohtsuka T, et al. Visual and semiquantitative analyses for F-18 fluorodeoxyglucose PET
scanning in pulmonary nodules 1 cm to 3 cm in size. Ann Thorac Surg 2005;
79: 984–988.
22. Herder G, Golding R, Hoekstra O, et
al. The performance of 18F-fluorodeoxyglucose
positron emission tomography in small solitary pulmonary nodules. Eur J Nucl Med Mol Imaging 2004; 31: 1231–1236.
23. Yaichiro H, Tetsuya T, Chisato K, et al. Accuracy
of PET for Diagnosis of Solid Pulmonary Lesions with 18F-FDG Uptake below
the Standardized Uptake Value of 2.5. Journal of Nuclear Medicine 2006; 47(3):
426-431
24. Demura Y, Tsuchida T, Ishizaki T, et
al. 18F-FDG
accumulation with PET for differentiation between benign and malignant lesions
in the thorax. J Nucl Med 2003; 44: 540–548.
25. Kumar
R, Loving V, Chauhan A, et al. Potential of Dual-Time-Point Imaging to Improve Breast
Cancer Diagnosis with 18F-FDG PET. J Nucl Med 2005; 46: 1819-1824.
26. Lodge M, Lucas J, Marsden P, et al.
A PET study of 18FDG
uptake in soft tissue masses. Eur J Nucl Med1999; 26: 22–30.
27. Hickeson M, Yun M, Matthies A, et
al. Use of a corrected
standardized uptake value based on the lesion size on CT permits accurate
characterization of lung nodules on FDG-PET. Eur J Nucl Med Mol Imaging 2002; 29: 1639–1647.
28. Dewan N, Shehan C, Reeb S, et al.
Likelihood of malignancy in a
solitary pulmonary nodule: comparison of Bayesian analysis and results of
FDG-PET scan. Chest 1997; 112: 416–422.
29. Hamberg L, Hunter G, Alpert N, et
al. The dose uptake ratio
as an index of glucose metabolism: useful parameter or oversimplification? J
Nucl Med 1994; 35: 1308–1312.
30. Knight S, Delbeke D, Stewart J, et
al. Evaluation of
pulmonary lesions with FDG-PET. Chest 1996; 109: 982–988.
31. Kapucu L, Meltzer C, Townsend D, et
al. Fluorine -18- fluoro- deoxyglucose
uptake in pneumonia. J Nucl Med 1998; 39: 1267 –1269.