ABSTRACT
Objectives: To measure the tensile peel strength of different
types of luting cements and studies their effect on the adhesive bond quality
of resin-bonded bridges.
Methods: Six
cements were investigated; two chemically adhesive resin cements (Super-Bond
C&B and Panavia 21), one compomer cement (Dyract Cem), two resin-modified glass
ionomer cements (Fuji Plus and RelyX Luting), and one conventional glass
ionomer cement (Ketac Cem). The tensile peel strength was investigated by
bonding grit-blasted Ni/Cr alloy beams to a block of the same alloy using the
different types of luting cements (n = 20 for each cement), leaving half the
length of the beam free. Beams were pulled off the block with a peeling action
by applying a tensile load to the free end of the beam and load at which failure
occurs was recorded. All the fractured surfaces of the tested samples were
examined under a stereo zoom microscope.
Results: Data were analyzed using one-way analysis of variance
(ANOVA), which showed significant differences between the mean tensile peel
strength of the cements (P < 0.05). Tukey's pairwise comparisons showed that the mean
tensile peel strength (in Newton)
of Super-Bond (7.7) was significantly greater than Panavia 21 (6.1) as well as
all other luting cements. Ketac Cem gave the lowest value of TPS (2.4). The
mode of failure for all the tested cements was cohesive in nature.
Conclusion: Adhesive resin cements have the highest tensile peel
strength which may explain their good clinical performance in resin-bonded
bridges compared to other luting cements.
Key words: Luting cement, Resin-bonded bridge, Tensile
peel strength.
JRMS
December 2012; 19(4): 24-30
Introduction
The resin-bonded bridge (RBB) is a
conservative alternative to conventional fixed bridge for the replacement of
one or two lost teeth with a minimal preparation of the abutments.(1,2)
It provides good aesthetic results and has low cost. The primary disadvantage
of RBB is that the longevity of the prosthesis is less than that for conventional
prosthesis.(2,3) Improvements in the prosthesis design, preparation
design and adhesive bond strength enhance the survival rates of RBB but there
are still an unacceptable number of clinical failures mostly because of
debonding.(4,5)
Assessment of bonding for RBB is usually
studied by measuring tensile or shear bond strength. The general view is that
the higher the tensile bond strength, the higher would be the adhesive bond
quality. Degrange et al.(6)
found higher values of tensile bond strengths with Panavia Ex material bonded
to Ni/Cr alloy compared to Super-Bond C&B (70.7 MPa and 28.5 MPa
respectively). With retainers having a
surface area of 10mm², the tensile force required to cause debonding would have
to be about 280 N - 700 N, and such high loads are unlikely to occur
clinically, nevertheless, debonding of the RBB is a common mode of failure.(4,5)
Another surprising observation is that the RBB most frequently fails at the
resin-metal interface leaving a layer of resin on the enamel.(4,5)
This contrasts with the observation that the tensile bond strength of
resin-metal is generally higher than that of resin-enamel.(4)
The tensile peel strength (TPS) test as a
means of assessment of bonding of RBB was explored by Northeast et al.(7)
They proposed that the failures of RBB occur due to tensile peel stresses in
the adhesive layer. The loading conditions result in a peeling action at the
adhesive interface and this may provide a more probable explanation for failure
of RBB than measurement of tensile or shear bond strengths.(7)
The purpose of this study was to measure
the TPS of different types of luting cements bonded to Ni/Cr alloy and study
the effect on the adhesive bond quality.
Methods
Six cements were investigated (Table I);
two chemically adhesive resin cements (Super-Bond C&B and Panavia 21), one
compomer cement (Dyract Cem), two resin-modified glass ionomer cements (Fuji
Plus and RelyX Luting), and one conventional glass ionomer cement (Ketac Cem).
Twenty Ni/Cr alloy beams (Talladium-V, Talladium,
Bucks, UK) 22mm long, 5 mm wide and 0.5 mm thick were used. A 1mm diameter
central hole was drilled 1.5mm from one end of each beam. A 15 mm brass block
(20 blocks) with a Ni/Cr alloy base bonded to one of its surfaces was also used
in this study (Fig. 1). Ni /Cr beams and blocks were blasted with fresh 50μm
alumina grit, washed in distilled water in an ultrasonic cleaner for 5 minutes
and then air-dried before bonding with the luting cements.
Manufacturers’ specifications as to proper
mixing time, paste-to-paste and powder-to-liquid ratios were carefully followed
during mixing of luting cement. After mixing the luting cement, it was applied
to the fitting surface of the beam. The beam was aligned perpendicular to the
centre of the free edge of the Ni-Cr block such that a 10 mm length of the beam
was bonded to the block with the aid of an alignment jig.
A compressive load of 40 N was applied
vertically to the beam (at about the middle of the 10 mm bonded to the block)
during setting of the cement using a Lloyd universal testing machine to produce
consistent cementation procedure. Excess cement was removed. 20 samples were
made for each type of the tested cements.
The samples were stored in the dry air at room temperature and tested
after 24 hours.
The prepared samples were mounted in a
Lloyd tensile machine (Lloyds Instruments. UK) with the free end of the beam
perpendicular to, and in line with, the load cell (100N). Each beam was pulled
off the block by a small hook that engaged the hole on the free end of the beam
(Fig. 2) at a crosshead speed of 1mm/min and the force at failure was recorded.
Results
The mean TPS values and the standard
deviations (in Newton)
are as follows: Super-Bond C & B 7.7
+/- 1.4, Panavia 6.1 +/- 1.3, GC Fuji plus 5.1 +/- 0.7, Rely X
Luting 4.5 +/- 0.8, Dyract Cem 4.2 +/- 1.3, Ketac Cem 2.4 +/- 0.4.
Fractured surfaces were examined at 40 X
magnifications under stereo zoom microscope. The mode of failure of all the
tested cements was similar in the first 2mm and was cohesive in nature. The
crack initiation took place close to the adhesive-substrate where there are
high tensile peel stresses. The fracture then travelled close to the beam-adhesive
interface (Fig. 3) leaving most of the luting cements on the block surface (Fig.
4).
There was no exposure of the grit-blasted
beam surfaces that were cemented with Super-Bond C&B and Panavia for the
whole bonded area of the beam. The beams that were cemented with other luting
cements were covered with the cement in the first 2mm of bonded surface. For the remaining length of beam surfaces, some areas were covered with the cement while other areas showed exposed grit-blasted metal.
Table I: Product and manufacturer information of luting cements that were tested
Luting Cement
|
Type
|
Setting
Reaction
|
Manufacturer
|
Super-Bond C&B*
|
4-META +PMMA
Adhesive resin
|
Polymerization
|
Sun Medical Co.,
Moriyama, Shiga, Japan.
|
Panavia 21
|
MDP/ Bis-GMA
Adhesive resin
|
Polymerization
|
Kuraray Co, LTD,
Osaka, Japan.
|
Dyract Cem Plus
|
Polyacid-modified
composite resin.
|
Polymerisation.
|
Dentsply DeTrey,
Konstanz, Germany.
|
RelyX Luting
|
Resin Modified
Glass Ionomer
|
Polymerisation
and Acid-Base reaction.
|
3M ESPE, St Paul, MN,
USA.
|
GC Fuji Plus
|
Resin Modified
Glass Ionomer
|
Polymerisation and
Acid-Base reaction.
|
GC America,
Chicago, III
|
Ketac Cem Aplicap
|
Conventional
Glass Ionomer
|
Acid-Base
reaction.
|
3M ESPE, St Paul, MN,
USA.
|
* This cement is also marketed by Parkell products (Farmington, NY,
Japan) under
the trade name C&B Metabond.
Table II: One-way analysis of variance for tensile peel
strength of all tested cements
Source
|
DF
|
SS
|
MS
|
F
|
P-value
|
Resin
|
5
|
319.90
|
63.98
|
55.96
|
0.000
|
Error
|
114
|
130.34
|
1.14
|
|
|
Total
|
119
|
450.25
|
|
|
|
Data were analyzed using one-way analysis
of variance (ANOVA), which showed significant differences between the mean TPS
of the cements (P < 0.05) (Table II). Tukey's pairwise comparisons (Fig. 5) showed that the mean TPS of
Super-Bond was significantly greater than Panavia as well as all other luting
cements. The mean TPS of Dyract Cem, Fuji Plus and RelyX Luting were not
significantly different. The
mean TPS of Ketac Cem was significantly the lowest.
Discussion
The RBB requires reliable bonding between
the tooth substrate and the cast metal framework. Assessment of bonding for RBB
is usually studied by measuring tensile or shear bond strength of resin-metal
bond and resin-enamel bond. The reported bond strengths of resin to enamel are
generally less than that of the resin to metal.(8) This would
indicate that resin- enamel bond is the weakest in this system and failure at
this interface should be most common. A surprising observation is that the RBB
most frequently fails at the resin-metal interface leaving a layer of resin on
the enamel.(4,5)
The concept of a TPS as a means of
comparing the adhesive capabilities of luting cements of RBB was explored by
Northeast et al.(7) They proposed that the loading
conditions, resulting in a peeling action at the adhesive interface, may
provide a more probable explanation for failure of RBB than measurement of
tensile or shear bond strengths.(7)
The TPS of different luting cements were
measured in this study. The experimental apparatus used was similar to that
used by Northeast et al.(7) The design of the TPS
experimental apparatus is somewhat similar to the design of the RBB if we
assume that the Ni-Cr beam acts as the retainer of RBB, the block as the tooth
structure and the pull out load as the load responsible for failure of RBB. The
TPS experimental apparatus is structural dependent as it depends on the beam
thickness; the thicker the beam the higher the TPS value obtained.(7)
With thicker retainer the level of stress within the luting cement was reduced,
showing that the stress the luting cement has to withstand is an important
contributory factor to the clinical outcome and is governed by enhancing the
mechanical properties of the luting cements. That means the values of TPS for
the luting cements used in this study will change if we change the thickness of
the beams. However, the 0.5 mm beam thickness is similar to the recommended
retainer thickness used clinically.
The TPS of the adhesive resin cements
(Super-Bond and Panavia) were significantly higher than all other cements.
Adhesive resin cements form chemical bonds
with clean sand blasted base metal surfaces.(9,10) The chemical
bonding of those resins to metal surface is facilitated by the high affinity of carboxylic monomer (found in
Super-Bond) and phosphate monomer (found in Panavia) to the oxide film found on
chromium containing non-precious alloys.(9,10) The TPS of
Super-Bond is significantly greater than that of Panavia. This may be due to
differences in the chemical composition of those materials; Panavia 21 is
Bis-GMA-based resin cement that contains high volume fraction of inorganic fillers. While Super-Bond is an unfilled
poly methyl methacrylate (PMMA) based resin cement that contains long flexible
chains of high molecular weight. Plastic deformation of the long flexible chains
delays the onset of brittle fracture, resulting in higher fracture toughness
value(11,12) and also higher TPS value.
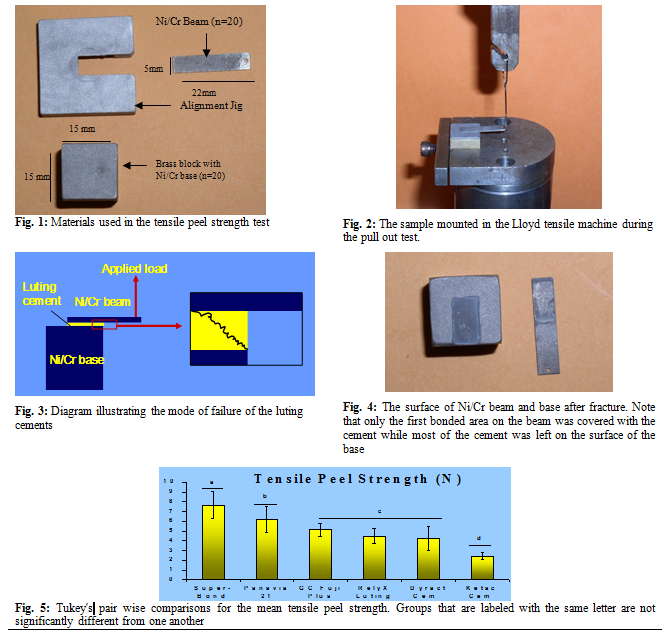
The TPS of compomer and resin-modified
glass ionomer lie between that of composite and glass ionomer. This is expected
as those materials have composition which lies somewhere on the continuum
between resin cements and glass ionomer cements. There was no significant
difference between the TPS of Dyract cem, Fuji Plus and RelyX Luting. This is
due to the relatively close chemical composition of those materials. The
presence of resinous components in compomer and resin-modified glass ionomer cements
has been shown to increase their fracture toughness.(12,13,14)
This could be responsible for the greater TPS than that of conventional glass
ionomer cements. The lower TPS of Dyract Cem (compomer) compared to Fuji Plus capsules
(resin-modified glass ionomer cements), although its not significantly
different, may be due to high voids incorporated during mixing of this powder/
liquid cement that cause stress concentration and lead to easier fracture.
Another factor may be the presence of urethane dimethacrylate (UDMA) in the
contents of Fuji Plus, which have been shown to increase the toughness of
composite materials.(15)
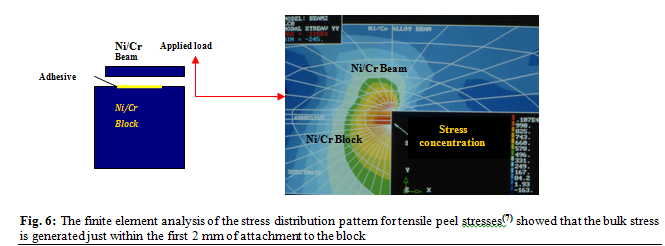
The TPS of Ketac Cem, conventional glass
ionomer cement, is significantly lower than all the other luting cements. Glass
ionomer cement is susceptible to dehydration and crazing during the initial
setting reaction.(12,13,15) The resultant microcracks would
act to initiate and facilitate crack propagation within the cement matrix.(12,13,15)
Clinically it may be advisable to protect the margins of the prostheses
cemented with glass ionomer with a protecting agent to avoid the dehydration
and crazing.
The TPS values obtained in this study are
generally lower than those obtained from tensile bond strength test, this
agrees with Northeast study.(7) Therefore, the load required
for bond failure to occur due to tensile peel stresses within the adhesive
interface is potentially more clinically relevant than measurement of tensile
or shear bond strengths, which
would predict higher failure loads that are unlikely to be encountered
clinically. The
reason for the high differences between tensile bond strength and TPS values
becomes clear when one considers how the load is distributed in the adhesive
layer for these different test arrangements. In the case of the tensile bond strength test, consisting of two rods
bonded together with the cement, the stress distribution in the cement layer is
relatively uniform.(16,17) This is not the case in the
tensile peel strength test where a large stress concentration is generated
within the first 2mm in the cement layer close to the free surface such that
the bulk of the cement layer is not being stressed and the rest of the retainer makes virtually no
contribution to its retention (Fig. 6).(7)
The standard deviation of the TPS test is
lower than that of the tensile bond strength tests which generally give high
standard deviations with coefficients of variations of more than 30%.(16,17)
In both tests the adhesive
layer has a large range of defects but in the tensile bond strength test the
failure is dictated due to defects in the total volume of the adhesive because
the adhesive is stressed everywhere. While in the tensile peel strength test,
the failure starts within a specific area of the adhesive layer such that the
distribution of defects within the rest of adhesive layer is ignored.
Generally, when the specimens bonded with
the cement were subjected to forces, the fracture initiated at the weaker
location in the specimen. In this study the mode of failure of all the studied
cements was virtually identical; this was cohesive in nature leaving most of
the cement on the substrate (Fig. 4). This is similar to the clinical failure
where most of the cement is left on the tooth surface after debonding.(4,5)
Since the fracture started from within
the cement itself and not at the beam-cement interface, this means it is the ability
of the cement to resist crack propagation or the fracture toughness of the
cement itself that may be responsible for the higher TPS of adhesive resin
cements compared to other luting cements. The TPS test is somewhat similar to
the fracture toughness test principle in that both of them measure the ability of
the material to resist crack propagation. That’s why it is not surprising to
find relationship between the fracture toughness and the TPS, such that the
material with higher fracture toughness has higher TPS.(18)
The high fracture toughness and TPS of the adhesive resin cements may explain
their good clinical performance in RBB. On the other hand, glass ionomer cement
showed unacceptable rate of debonding although it adheres well to tooth
structure and metals.(19,20) This could be due to their low fracture
toughness and TPS which may contraindicate their use in RBB. Studies indicated that the problem with the
glass ionomer cements is a lack of strength of the material itself, not of the
adhesive bond to the substrate.(19,20)
But this does not mean that the fracture
toughness of the luting cement is the only property that is needed to enhance
the outcome of the RBB. Other mechanical properties of the luting cement are
still important. We still need a material with a high diametral tensile and
compressive strength in order to resist stresses within the adhesive layer and
tolerate the masticatory forces.(21,22) Elastic modulus is also important, it has been suggested that
luting cement with an elastic modulus in the intermediate range between that of
tooth structure and the indirect restorative material is desirable because this
can reduce interfacial stress concentrations without causing excessive strains.(21,22)
The tests used to assess the adhesive resin
cements of RBB give contradictory results. The tensile bond strength test
showed higher values for Panavia compared to Super-Bond.(6,22,23) On the other hand TPS values
obtained in this study showed higher values for Super-Bond compared to Panavia.
The wedge test showed higher fracture energy of Super-Bond compared to
Panavia.(6,22,23) The fatigue tests showed that Super-Bond C&B resin cement had an inferior
fatigue bond strength compared to Panavia and Comspan (Bis-GMA resin cement)
bonded to different types of alloy.(24-26) There is a need to establish which test is
more appropriate in order to determine which type of adhesive will give better
clinical performance.
Especially for RBB, the TPS and the
fracture toughness data may provide better information than standard tensile
bond strength data upon the behaviour of adhesive joints under clinical
conditions. In the oral cavity, however, it is considered that factors such as
saliva, cyclic occlusal loading, and thermal stresses during function will
affect the luting cement and bonding interface over time. So further studies
under extensive and prolonged laboratory aging conditions that better simulate
clinical situation are needed before judging the higher performance of one
material over other materials.
Conclusion
Within the limitations of this study it is concluded
that:
· Adhesive resin cements have
the highest TPS which may explain their good clinical performance in RBB
compared to other luting cements. Among the adhesive resin cements, Super-Bond
had higher TPS than Panavia 21.
· TPS data for cements may provide
a more probable explanation for failure of RBB compared to tensile bond
strength data which would predict higher failure loads that are unlikely to be
encountered clinically.
Acknowledgements
Many thanks and deep appreciation to Prof.
Richard van Noort for his invaluable guidance and help that he gave throughout
the whole study.
References
1.Hussey DL, Pagni C, Linden GJ. Performance of
400 adhesive bridges fitted in a restorative dentistry department. J Dent
1991; 19: 221-225
2.El-Mowafy O, Rubo MH. Resin-bonded
fixed partial dentures-A literature review with presentation of a novel
approach. Int J Prosthodont 2000; 13: 460-467.
3.Djemal S, Setchell D, King P,
Wickens J. Long-term survival characteristics of 832
resin-retained bridges and splints provided in a post-graduate teaching
hospital between 1978 and 1993. J Oral Rehabil 1999; 26: 302-320
4.Wyatt CC. Resin-bonded fixed partial dentures: what's new? J Can Dent Assoc 2007; 73 (10):933-8.
5.Creugers NH,
Kayser AF. An analysis of multiple failures of resin bonded
bridges. J Dent 1992; 20: 348-351.
6.Degrange M, Edward V,
Toumelin JP. Comparison of two testing methods for resin-bonded
bridges. J Dent Res 1991; 70: 741. [Abstract]
7.Northeast SE, van Noort R,
Shaglouf AS. Tensile peel failure of resin-bonded Ni/Cr beams:an
experimental and finite element study. J Dent 1994; 22: 252-256.
8.Livaditis GJ, Thompson VP. Etched castings:
an improved retentive mechanism for resin-bonded retainers. J Prosthet Dent
1982; 47: 52-58.
9.Tanaka T, Nagata K, Takeyama
M, et al. 4-META opaque resin--a
new resin strongly adhesive to nickel-chromium alloy. J Dent Res 1981;
60:1697-706.
10.Atta
MO, Smith BG, Brown D. Bond strengths
of three chemical adhesive cements adhered to a nickel-chromium alloy for
direct bonded retainers. J Prosthet Dent 1990; 63: 137-143.
11.Johnson WW, Dhuru VB, Brantley WA. Composite
microfiller content and its effect on fracture toughness and diametral tensile
strength. Dent Mater 1993; 9: 95-
98
12.Knobloch LA, Kerby RE, Seghi R, et al. Fracture
toughness of resin-based luting cements. J Prosthet Dent 2000; 83:
204-209.
13. Ryan AK, Orr JF, Mitchell CA. A comparative
evaluation of dental luting cements by fracture toughness tests and
fractography. Proc Inst Mech Eng 2001; 215:65-73.
14.Cook WD, Moopnar M. Influence of chemical
structure on the fracture behaviour of dimethacrylate composite resins. Biomaterials
1990; 11: 272-276.
15. Mitchell CA, Douglas
WH, Cheng YS. Fracture toughness of conventional,
resin-modified glass-ionomer and composite luting cements. Dent Mater 1999;
15: 7-13
16.Van Noort R, Noroozi S, Howard IC, Cardew G. A critique of
bond strength measurements. J Dent 1989; 17: 61-67.
17.Van Noort R, Cardew GE, Howard IC, Noroozi S. The effect of
local interfacial geometry on the measurement of the tensile bond strength to
dentin. J Dent Res 1991; 70: 889-893.
18.Van Noort R, Northeast SE, El-Masmari. The tensile peel
strength of a toughened adhesive. J Dent Res 1995; 74: 981.
[Abstract]
19.McComb D, Sirisko R, Brown J. Retention of
castings with glass ionomer cement. J Prosthet Dent 1984;
48:285-288
20.Hibino Y, Kuramochi K, Hoshino T, et al. Relationship
between the strength of glass ionomers and their adhesive strength to metals. Dent
Mater 2002; 18: 552-557
21.Li ZC, White SN. Mechanical properties of
dental luting cements. J Prosthet Dent 1999; 81: 597- 609.
22.Rosenstiel SF, Land MF, Crispin BJ. Dental luting
agents: A review of the current literature. J Prosthet Dent 1998; 80:
280-301.
23.Degrange M, Roulet JF. Minimally
invasive restorations with bonding. Quintessence books 1997. Chapter 9-13.
Pages 153-177.
24.Degrange M, Charrier JL, Attal JP, Asmussen E. Bonding of
luting materials for resin-bonded bridges: clinical relevance of in vitro
tests. J Dent 1994; 22 Suppl 1:S28-32
25.Aquilino SA, Diaz-Arnold AM, Piotrowski TJ. Tensile fatigue
limits of prosthodontic adhesives. J Dent Res 1991; 70: 208-210.
26.Fitchie JG, Zardiackas LD,
Givan DA, et al. Tensile fatigue of two composite cements
bonding three base metal alloys to bovine enamel. Dent Mater 1993; 9:
28-32.